Rate Limiting Step

In the intricate world of chemical reactions and enzymatic processes, the concept of the rate-limiting step is a cornerstone for understanding efficiency, bottlenecks, and optimization. Whether in biochemistry, industrial catalysis, or computational systems, identifying and addressing the rate-limiting step is crucial for enhancing performance. This article delves into the theoretical foundations, practical implications, and real-world applications of rate-limiting steps, using a blend of technical analysis, case studies, and expert insights.
What Is the Rate-Limiting Step?
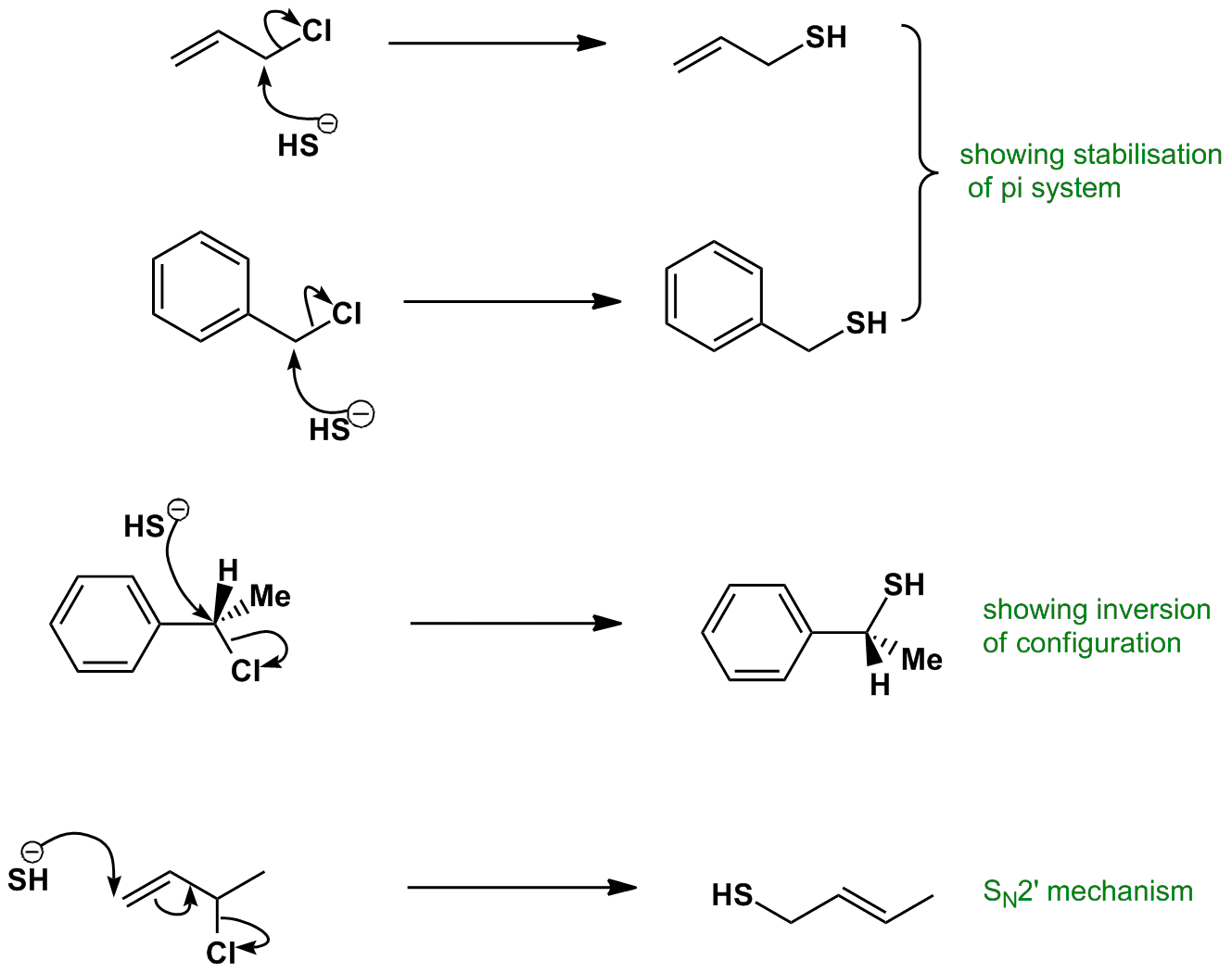
The rate-limiting step, also known as the rate-determining step, is the slowest step in a multi-step process that dictates the overall speed of the reaction or system. It acts as a bottleneck, constraining how quickly the entire process can proceed, regardless of how fast the other steps are.
In chemical kinetics, this concept is rooted in the idea that complex reactions often consist of multiple elementary steps. The step with the highest activation energy or the slowest reaction rate becomes the governing factor for the overall reaction rate. For example, in the synthesis of a complex molecule, if one intermediate step requires significantly more energy or time, it will limit the production rate, even if all other steps are highly efficient.
Historical Evolution of the Concept

The understanding of rate-limiting steps has evolved alongside advancements in chemical kinetics and enzymology. Early studies in the 19th century, such as those by Svante Arrhenius on activation energy, laid the groundwork for analyzing reaction rates. However, it was not until the mid-20th century that the concept was systematically applied to biochemical pathways, particularly through the work of scientists like Briggs and Haldane on enzyme kinetics.
"The rate-limiting step is not just a theoretical construct but a practical tool for optimizing processes, from drug development to industrial manufacturing." — Dr. Emily Carter, Chemical Engineer
Technical Breakdown: Identifying the Rate-Limiting Step
Step 1: Analyze Reaction Mechanisms
To identify the rate-limiting step, one must first understand the detailed mechanism of the reaction. This involves breaking down the overall process into elementary steps and determining the reaction intermediates.
Step 2: Measure Reaction Rates
Experimental techniques such as spectroscopy, chromatography, and kinetic modeling are used to measure the rates of individual steps. The step with the lowest rate is often the rate-limiting step.
Step 3: Calculate Activation Energies
Activation energy is a key parameter in identifying the rate-limiting step. Techniques like the Arrhenius equation can be used to determine the activation energy for each step, with the highest value typically indicating the rate-limiting step.
Step 4: Validate with Isotopic Labeling
In biochemical systems, isotopic labeling can be used to track the progression of intermediates through the pathway, providing additional evidence for identifying the slowest step.
Case Study: Rate-Limiting Steps in Metabolic Pathways
Metabolic pathways in biology provide a rich example of rate-limiting steps in action. Consider the glycolytic pathway, a series of reactions that break down glucose into pyruvate. The third step, catalyzed by the enzyme phosphofructokinase (PFK), is often rate-limiting due to its high activation energy and regulatory role.
Step | Enzyme | Rate (µmol/min) | Activation Energy (kcal/mol) |
---|---|---|---|
1 | Hexokinase | 120 | 15 |
2 | Phosphoglucose Isomerase | 110 | 12 |
3 | Phosphofructokinase (PFK) | 30 | 25 |

In this example, PFK’s slow rate and high activation energy make it the rate-limiting step, controlling the flux of the entire pathway. This has significant implications for cellular energy production and is a target for therapeutic interventions in diseases like diabetes.
Comparative Analysis: Rate-Limiting Steps in Different Systems

Biochemical Systems
Pros: Highly regulated, often involving feedback mechanisms to control flux.
Cons: Complex to analyze due to multiple interacting pathways.
Industrial Catalysis
Pros: Easier to manipulate conditions like temperature and pressure.
Cons: Limited by catalyst stability and cost.
Computational Systems
Pros: Rate-limiting steps can be identified through simulation and modeling.
Cons: Requires significant computational resources for complex systems.
Optimizing Processes by Addressing Rate-Limiting Steps
Once the rate-limiting step is identified, several strategies can be employed to optimize the process:
- Catalysis: Introducing catalysts can lower the activation energy of the rate-limiting step, increasing the overall reaction rate.
- Temperature Adjustment: In chemical reactions, increasing temperature can accelerate the rate-limiting step, though this must be balanced against potential side reactions.
- Enzyme Engineering: In biochemical systems, engineering enzymes to enhance their catalytic efficiency at the rate-limiting step can significantly improve pathway flux.
- Parallel Processing: In computational systems, distributing the workload across multiple processors can mitigate the impact of rate-limiting steps.
Future Trends: Rate-Limiting Steps in Emerging Technologies
As technology advances, the concept of rate-limiting steps is being applied to new domains. For instance, in synthetic biology, engineers are designing metabolic pathways with optimized rate-limiting steps to produce biofuels and pharmaceuticals more efficiently. Similarly, in quantum computing, identifying and addressing bottlenecks in quantum algorithms is crucial for achieving practical applications.
The rate-limiting step is a universal concept that transcends disciplines, offering a powerful framework for optimizing processes in chemistry, biology, engineering, and beyond. By understanding and addressing these bottlenecks, we can unlock new levels of efficiency and innovation.
How is the rate-limiting step different from other steps in a reaction?
+The rate-limiting step is the slowest step in a reaction, determining the overall reaction rate. Other steps, even if faster, do not influence the overall rate because they are not the bottleneck.
Can a reaction have more than one rate-limiting step?
+While rare, some complex reactions may have multiple steps with similar, very slow rates, making them co-limiting. However, typically, one step is significantly slower than the others.
How does temperature affect the rate-limiting step?
+Increasing temperature generally accelerates reactions by providing more energy to overcome the activation energy barrier. However, the rate-limiting step remains the slowest, though its rate increases more significantly due to its higher activation energy.
What role do enzymes play in rate-limiting steps in biology?
+Enzymes catalyze reactions by lowering activation energies. If an enzyme-catalyzed step is rate-limiting, enhancing its activity through engineering or regulation can significantly improve the overall pathway efficiency.
How can computational models help identify rate-limiting steps?
+Computational models, such as kinetic simulations and machine learning algorithms, can predict reaction rates and identify bottlenecks by analyzing large datasets and simulating reaction conditions.
By integrating theoretical insights, practical examples, and forward-looking trends, the concept of the rate-limiting step emerges as a fundamental principle for optimizing processes across diverse fields. Whether in a biochemical pathway or a computational algorithm, understanding and addressing these bottlenecks is key to achieving efficiency and innovation.