H2 Mo Diagram Understand Bonding Easily
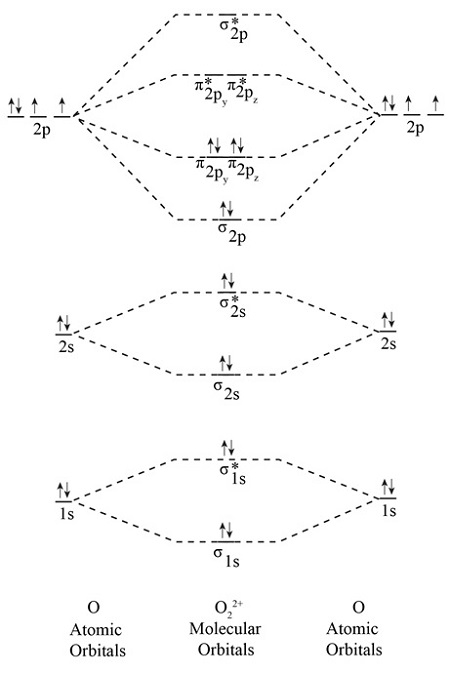
Understanding Chemical Bonding Through the H₂ Molecular Orbital (MO) Diagram
Chemical bonding is the cornerstone of chemistry, dictating the structure, properties, and reactivity of molecules. Among the simplest yet most instructive examples is the hydrogen molecule (H₂). By analyzing its molecular orbital (MO) diagram, we gain profound insights into the nature of covalent bonding. This article breaks down the H₂ MO diagram, exploring its theoretical foundations, practical implications, and broader significance in chemistry.
The Theoretical Framework: Molecular Orbital Theory
Molecular Orbital (MO) theory is a quantum mechanical model that describes the behavior of electrons in molecules. Unlike Valence Bond Theory, which focuses on atomic orbitals overlapping, MO theory constructs new orbitals—molecular orbitals—that encompass the entire molecule. These orbitals are formed by the linear combination of atomic orbitals (LCAO), resulting in bonding, antibonding, and nonbonding MOs.
For H₂, the interaction of two 1s atomic orbitals (from each hydrogen atom) generates two molecular orbitals:
1. σ (sigma) bonding orbital (σ₁s): Lower in energy, promotes bonding by concentrating electron density between nuclei.
2. σ* (sigma star) antibonding orbital (σ*₁s): Higher in energy, reduces bonding by placing electron density away from nuclei.
Step-by-Step Analysis of the H₂ MO Diagram
Comparative Analysis: H₂ vs. He₂
Molecule | Electron Configuration | Bond Order | Stability |
---|---|---|---|
H₂ | σ₁s² | 1 | Stable |
He₂ | σ₁s² σ*₁s² | 0 | Unstable |

This comparison highlights the critical role of bond order. While H₂ has a bond order of 1, He₂’s bond order of 0 explains its non-existence under normal conditions.
Historical Evolution of MO Theory
MO theory emerged in the early 20th century, pioneered by Friedrich Hund, Robert Mulliken, and John Lennard-Jones. Its development was spurred by the need to explain phenomena like paramagnetism in O₂, which Valence Bond Theory couldn’t address. The H₂ MO diagram became a foundational example, demonstrating the theory’s predictive power.
Practical Applications and Implications
- Catalysis: Hydrogenation reactions rely on H₂’s bonding properties, critical in industries like petroleum refining.
- Materials Science: Hydrogen bonding in polymers influences mechanical properties, as seen in nylon or Kevlar.
- Astrophysics: H₂ is the most abundant molecule in the universe, shaping star formation and interstellar chemistry.
Myth vs. Reality: Common Misconceptions
Future Trends: MO Theory in Computational Chemistry
Advances in computational methods, such as Density Functional Theory (DFT), leverage MO concepts to model complex systems. For instance, designing hydrogen storage materials requires understanding H₂ bonding at atomic scales. Future applications may include:
- Quantum Computing: Simulating MO interactions for drug discovery.
- Green Energy: Optimizing hydrogen fuel cell catalysts.
Key Takeaways
Why does H₂ have a bond order of 1?
+H₂ has two electrons in the σ₁s bonding orbital and none in the σ*₁s antibonding orbital. Bond order = (2 – 0) / 2 = 1, indicating a single covalent bond.
What happens if the σ*₁s orbital is occupied?
+If the σ*₁s orbital is occupied (as in He₂), the bond order becomes 0, making the molecule unstable and non-existent.
How does MO theory differ from Valence Bond Theory?
+MO theory treats electrons as delocalized over the entire molecule, forming new molecular orbitals. Valence Bond Theory focuses on localized atomic orbital overlap.
By dissecting the H₂ MO diagram, we not only demystify chemical bonding but also unlock tools to innovate across science and technology. Its elegance lies in its simplicity—a single molecule revealing universal principles.