Aromatic Vs Antiaromatic Vs Nonaromatic

In the realm of organic chemistry, the concepts of aromaticity, antiaromaticity, and nonaromaticity are fundamental to understanding the stability, reactivity, and electronic structure of cyclic compounds. These terms describe the unique electronic properties of molecules, particularly those containing conjugated π-electron systems. Let’s delve into the fascinating world of these chemical phenomena, exploring their definitions, characteristics, and implications.
Unraveling the Mystery of Aromaticity

Aromaticity is a property that bestows exceptional stability upon certain cyclic compounds, making them less reactive than their non-aromatic counterparts. This concept is deeply rooted in the behavior of electrons within these molecules.
The Birth of Aromaticity: A Historical Perspective
The term “aromatic” originally referred to the pleasant odors of certain compounds, such as benzene. However, modern chemistry has redefined aromaticity as a specific set of electronic characteristics. In 1865, August Kekulé proposed the cyclic structure of benzene, a breakthrough that laid the foundation for understanding aromaticity.
Defining Aromatic Compounds
Aromatic compounds, or aromatics, are cyclic, planar molecules with a continuous ring of overlapping p-orbitals, resulting in a closed loop of π-electrons. These electrons are delocalized, meaning they are not confined to a single bond or atom but are spread across the entire ring. This delocalization leads to several key characteristics:
Hückel’s Rule: A fundamental principle in aromaticity, Hückel’s Rule states that a compound is aromatic if it has (4n + 2) π-electrons, where n is a non-negative integer (0, 1, 2, …). This rule predicts the stability and aromatic nature of compounds like benzene (n = 1, 6 π-electrons).
Planarity: Aromatic molecules are typically planar, allowing for maximum overlap of p-orbitals and efficient delocalization of π-electrons.
Complete Conjugation: All atoms in the ring must be capable of p-orbital overlap, ensuring a continuous electron cloud.
Stability: Aromatic compounds exhibit unusual stability, often resisting reactions that would disrupt their aromatic system.
Antiaromaticity: The Flip Side of the Coin
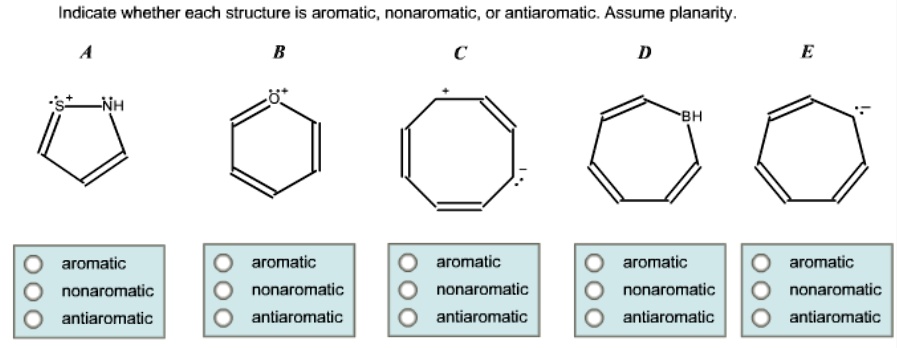
Antiarromaticity is the opposite of aromaticity, representing a class of compounds that are highly unstable due to their electronic structure.
Characteristics of Antiaromatic Compounds
Hückel’s Rule Violation: Antiaromatic compounds have (4n) π-electrons, which leads to a destabilized electronic configuration.
Planarity and Conjugation: Like aromatics, antiaromatics are planar and fully conjugated, but their electron count results in a higher energy state.
Instability: These compounds are highly reactive and tend to undergo reactions that relieve the strain caused by their antiaromaticity.
Nonaromatic Compounds: The Middle Ground
Nonaromatic compounds occupy the space between aromatic and antiaromatic systems, lacking the specific electronic characteristics of either.
Features of Nonaromaticity
Electron Count: Nonaromatic compounds do not follow Hückel’s Rule and can have any number of π-electrons.
Structural Flexibility: They may be planar or non-planar and do not require a fully conjugated system.
Reactivity: Nonaromatic compounds exhibit typical reactivity patterns, neither exceptionally stable nor unstable.
Comparative Analysis: Aromatic vs. Antiaromatic vs. Nonaromatic
Characteristic | Aromatic | Antiaromatic | Nonaromatic |
---|---|---|---|
Hückel’s Rule | (4n + 2) π-electrons | (4n) π-electrons | Any π-electron count |
Stability | Highly stable | Highly unstable | Typical stability |
Planarity | Planar | Planar | Planar or non-planar |
Conjugation | Fully conjugated | Fully conjugated | May or may not be conjugated |
Reactivity | Resists reactions disrupting aromaticity | Undergoes reactions to relieve strain | Typical reactivity |

The Role of Molecular Orbital Theory
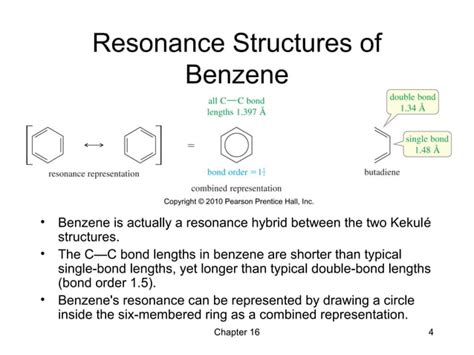
Molecular Orbital (MO) theory provides a powerful framework for understanding aromaticity and antiaromaticity. In this theory, the π-electrons in a cyclic compound occupy molecular orbitals, which can be visualized as a set of energy levels.
Aromaticity and MO Theory
In aromatic compounds, the (4n + 2) π-electrons fill the bonding molecular orbitals, resulting in a stable, lower energy configuration. This is often represented as a set of nested, energy-level diagrams, with electrons occupying the lower energy levels.
Antiaromaticity in MO Theory
Antiaromatic compounds, with their (4n) π-electrons, have a different MO configuration. The electrons occupy both bonding and antibonding orbitals, leading to a higher energy state. This destabilization is a key factor in their reactivity.
Practical Implications and Applications
Understanding aromaticity, antiaromaticity, and nonaromaticity has significant practical implications in various fields:
Pharmaceuticals: Many drugs contain aromatic rings, which contribute to their stability and bioactivity.
Materials Science: Aromatic compounds are used in polymers and materials, providing strength and stability.
Organic Synthesis: Chemists use aromaticity principles to design and predict reaction outcomes.
Environmental Chemistry: Aromatic compounds are often involved in environmental processes, such as pollutant degradation.
Addressing Common Misconceptions
Future Trends and Research Directions
The study of aromaticity continues to evolve, with ongoing research exploring:
- Expanded Aromaticity: Investigating larger, more complex aromatic systems.
- Bioaromaticity: Understanding aromaticity in biological molecules and its role in biological processes.
- Computational Methods: Developing advanced computational tools to predict and analyze aromaticity in complex molecules.
FAQ Section
How does aromaticity affect chemical reactions?
+Aromatic compounds often resist reactions that would disrupt their aromatic system, leading to unique reactivity patterns. For example, benzene undergoes substitution reactions preferentially over addition reactions.
Can a compound be both aromatic and antiaromatic?
+No, a compound cannot simultaneously exhibit both aromatic and antiaromatic properties. These are mutually exclusive electronic states.
What is the role of conjugation in aromaticity?
+Conjugation is essential for aromaticity as it allows for the delocalization of π-electrons across the ring. This delocalization is a key factor in the stability of aromatic compounds.
How do chemists determine if a compound is aromatic?
+Chemists use a combination of Hückel's Rule, molecular orbital theory, and experimental observations (e.g., NMR spectroscopy) to determine aromaticity.
Are there any real-world applications of antiaromatic compounds?
+While antiaromatic compounds are often unstable, their reactivity can be harnessed in synthetic chemistry. For instance, cyclobutadiene derivatives can be used as intermediates in organic synthesis.
In conclusion, the concepts of aromaticity, antiaromaticity, and nonaromaticity are fundamental to organic chemistry, providing insights into the stability, reactivity, and electronic structure of cyclic compounds. From the historical origins of aromaticity to its modern applications, this field continues to captivate chemists and drive innovation. As research progresses, we can expect further revelations about the intricate world of aromatic systems and their impact on various scientific disciplines.